Influence of the Preheating on the Thermal Transient State in Coating Welding on AISI 1045 Steel: Microstructure and Microhardness
Received Date: August 08, 2020 Accepted Date:August 21, 2020 Published Date:August 24, 2020
doi: 10.17303/jmsa.2020.4.202
Citation:Maria Cristina Carrupt Ferreira Borges (2020) Influence of the Preheating on the Thermal Transient State in Coating Welding on AISI 1045 Steel: Microstructure and Microhardness. J Mater sci Appl 4: 1-7.
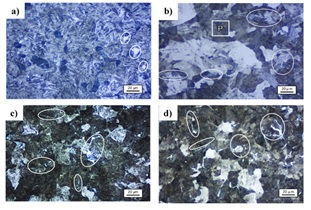
Abstract
In an electric arc welding process, the heat distribution through the material at the beginning of the welding it is not homogeneous. This condition is known as a transient state. In theory, after a period of time and a certain distance it becomes uniform, meaning that the quasi-stationary state has been reached. The heat flow during the welding process has an important hole about phases transformations and consequentially about resulting microstructure and materials mechanical properties. The heat flow in welding depends on the thermal variables as thermal diffusivity, thermal conductivity, initial temperature, etc. and it directly affects the metallurgical transformations of material. The present work has aimed to study if the preheating applied for coating welding affects the transient state, once that the quasi-stationary state only achieves under specifics conditions. The base material used was AISI 1045 steel and the coating was deposited using submerged arc welding (SAW). The preheating temperature was calculated considering both chemical composition and thickness of base material. In order to analyses the welded joint, a light microscope was utilized just as microhardness (Vickers). The results show that the preheating and the thickness of the plate influenced the transient state.
Keywords: Transient state; Preheating; Microstructure; Microhardness
Introduction
All the electric arc welding process input on the material a thermal cycle. The material suffers a had heating in a few seconds until a very high temperature (peak temperature), followed by a quick cooling, without a time to homogenize the temperature throughout the base material. This is due to the fact that the heat source is not static [1-6]. The peak temperature depends on the distance between the measurement point and the heat source. The further away from the heat source, the lower the peak temperature value. Therefore, the thermal cycle is not the same on the material surface and each point has it's own [1-4]. They are responsible for the phase's transformation on solid-state and consequentially for the resultant microstructure when the peak temperature achieves a critical enough value for it. Thus, the properties of the material affect, just as the weld residual stress and mechanical distortion [1-5].
The movement of the heat source causes two different heat flow behaviors: the thermal transient state and the quasi-stationary state [1]. The thermal transient state occurs at the beginning of welding when the heat source is started and at the end when the heat source is extinguished. This state is characterized by a thermal imbalance where the temperature distribution is not uniform through the material [1]. After a period of time and a certain displacement of heat source, the material finds a thermal equilibrium where the temperature distribution becomes uniform, which is the quasi-stationary state [1, 2, 4]. The thermal transient state affects the material properties in a different way than the quasi-stationary state. Once that the quasi-stationary state only occurs under specifics conditions, to understand the transient state becomes important to get the best conditions of resulting microstructure and physical properties for the welded joint.
This work was developed in order to verify the effect of preheating on the thermal transient state. It is important because a decrease in this state means an increase in the period of thermal equilibrium, ensuring greater uniformity on the properties and microstructure. The analysis was made by comparing microstructures and microhardness in four different conditions.
Materials and Methods
This experimental work aims to evaluate the influence of preheating on the transient state. For this, a plate of AISI 1045, according to NBR NM 87/2000, with dimensions 200 x 100 x 25.4mm (length-width-thickness), was welding by SAW (Submerge Arc Welding) process, using a Lincoln Weld Solid State LT-7 Tractor, frequency inversion, Power Wave 1000. The welding consumables used were AWS F7AZ-EL12 and AWS EM12K. The seam was only deposited on the plate as in welding for coating.
The pre-heating was defined by equivalent carbon (CE) [1,9]. The aim of preheating is to decrease the risk of cold cracking induce by hydrogen [1,4,7,8]. The range of preheating temperature used in this work was 210°C - 230°C. The preheating was applied directly using an oxi-acetylene flame. The other parameters are: 500 – 600 A; 28 – 30 V; 0.365 cm/s.
To determine the theoretical limit between the end of the transient state and the start of the quasi-stationary state, the equations (1) and (2) was used [5]. The calculated limit was: r = 16.03 mm
Where: t = time to achieve the start of quasi-stationary state (s); vs = welding speed (cm/s); r = distance until the start of quasi-stationary state (cm); K = thermal diffusivity (cm2 /s) [5].
The thermal diffusivity was calculated with the equation (3) [1-4]. Where: λ = thermal conductivity (J/s.mm.°C); ρ = specific weight (g/mm3); Cp = specific heat (J/g °C).
The plate was prepared as showed in Figure 1. A line indicating the theoretical limit between the transient state and quasi-stationary state was drawn. Points for temperature measurement was made before and after this line, positioned 5 mm from the reference line.
The temperature was measured to plot the thermal cycle curves. The measurement was performed with a MINIPA Digital Thermometer MT-550 and a K-type penetration thermocouple, temperature range 200°C - 1370°C ± 2°C of accuracy. The temperature data were recorded every 10 seconds in an interval from 0 seconds to 900 seconds. Four seams were deposited. In the seam 1 and seam 3, the temperature measurement was made before the reference line to start the quasi-stationary state. At seam 2 and seam 4, the temperature measurement was made after the reference line to start the quasi-stationary state. The preheating has been done only in seams 3 and 4.
Microstructural and microstructural changes were analyzed by optical microscopy. Of each seam two specimens were taken, one before the theoretical limit and other after this, both distant 5mm way from this limit. The specimens were prepared according to the standard ASTM E 3-80. The macro etching and micro etching follow the standards ASTM E 340-87 and ASTM E 407-70, respectively. The reagent used was nital 2%.
The microhardness test was performed according to standards ASTM E 384-89 and ASTM A 370. The applied load was 0,5 kgf. Microhardness was measured through the welded joint, starting in base metal (BM), passing HAZ, and ending in welding metal (WM) zone, as shown in Figure 2. Two measurement lines were done on each specimen: Line 1next to the base material surface and Line 2 passing halfway through the penetration of weld metal. Three specimens of each seam were analyzed as follow: at 5 mm before the theoretical limit (specimen 1), on the theoretical limit (specimen 2) and other at 5 mm after theoretical limit (specimen 3), i.e. observation was made at 11 mm, 16mm and 21 mm, after opening electric arc.
Results and Discussion
Macroscopy
In this work, the macroscopy has been done on the transverse section of welded joints by etching with nital 2% for 12 seconds. The macroscopy results are shown in Figure 3. In a qualitative analysis of macrographs, is possible to observe that in image 3(a) the seam has bell format.
This format can drive to solidification cracks [1, 4]. Comparing images 3(a) and 3(b), we can see that in 3(b), the preheating promoted better format and an increase in HAZ extent. How the initial temperature is lower in 3(a) than in 3(b), the penetration extent of weld metal into the material base is less in 3(a) [9, 10]. CARVALHO, 2014 [11] also observed this phenomenon, joints welded with less heat input showed less penetration either.
Comparing image 3(b) with the images 3(c) and 3(d), it is possible to notice that there is a great similarity between then, about the seam format, penetration, and extent of HAZ. The preheating applied on 3(b) provided a heat flow similar to that obtained after the end of the transient state, i.e. a heat flow as in the quasi-stationary state. Thus, maybe it possible to think that the preheating has reduced the period of the transient state.
Microscopy
The next figures show images of micrographs with 500x of magnification. The etching was done by nital 2%, during 8seconds.
Figure 4 brings micrographs of base metal. The white regions with a well-defined boundary are ferreite (F) and the dark regions are perlite colonies (P). In 4(b) we can see that the heat flow at quasi-stationary state promoted the growth of primary ferrite regions and this microstructure is similar to those in the imagens 4(c) and 4(d) that have been preheated. The preheating decreases the cooling rate, favoring the growth of primary ferrite [2,12]. The heat flow at the quasi-stationary state has the same effect.
Figure 5 shows the micrographs of HAZ. In 5(a) the microstructure is predominantly bainitic [12,13]. The circled areas may be small regions of martensite. The absence of preheating drives to a cooling rate that results in a bainitic and martensitic transformation [1,2,4]. In 5(b), although this specimen hasn’t been preheated either, we can observe that there are little regions of primary ferrite and Widmanstatten Ferrite (circled areas). The microstructure is predominantly perlitic. It is possible because of when the quasi-stationary state is achieved, the heat flow comes to establish resulting in a lower cooling rate [4,14,15,16]. In both 5(c) and 5(d), the specimens were preheating. The resulting microstructure is composed of primary and Widmanstatten ferrites (circled areas) and perlite.
In a qualitative analysis, an increase in ferrite regions could be observed due to the decrease in the cooling rate because of the preheating [1,4,15,16]. Once that the microstructure observed in 5(c) is very similar to the microstructure has been observed in imagens 5 (b) and 5 (d), both obtained after the theoretical limit established, the hypothesis about the preheating having anticipated the end of transient state can be formulated.
Figure 6 brings imagens of WM. In 6(a), there is a predominance of acicular ferrite between thin primary ferrite on grain boundary, characteristic of high cooling rate [12]. The dark region could be identified as ferrite carbide aggregate (FC(P)), maybe perlite. In 6(b), because of a decrease on cooling rate after the end of transient state [2,5], there is an increase in regions of primary ferrite (PF), in the regions of Widmanstatten Ferrite, and an increase in regions of ferrite carbide aggregate (FC(P)) too. In 6(c) and 6(d), the preheating favored the development of primary ferrite (PF(G)), decreasing the regions of acicular ferrite (AF) [12]. On both, there are dark regions that are characteristics of (FC(P)). As the cooling rate in 6(d) is less than in 6(c), the development of primary ferrite was favored in 6(d) and no formation of FS(A) is observed.
Microhardness
Figure 7 shows the curves of microhardness obtained from each seam. The seam 1 and seam 2 haven't been preheated as opposed to seam 3 and seam 4.In general, the curves show that the highest hardness values are concentrated in the HAZ, because of the severe cooling rates at this region and the equivalent carbon either [10].
Analyzing the curves of specimen 1, noticed that the seam 1 and seam 2 have higher hardness values, for Line 1. These values are in the range of hardness relative to the martensitic microstructure [12], in agreement with the microstructure shown in Figures 8(a) e 8(b). The hardness values measured to seam 3 and seam 4 are below of this range, because of the preheating applied [15]. The curves of Line 2, still for specimen 1, shows a decrease in values of seam 2, probably due to the thickness influence on the heat flow [1, 3]. The hardness value of specimen 2 has been decreased either, but still remained in the martensitic range. At Line 2, the seams have very close values of hardness, once more time probably provided that the thickness influence [1, 3]. For specimen 3, was observed that there is no grate variation between the hardness values for the three regions, both on Line 1 and on Line 2, except to the seam 1 in Line 1. It could mean that the quasi-stationary state may have been reached [10]. Assuming that the microhardness of specimen 3 was measured 5 mm after the theoretical limit, it means that the quasi-stationary state started between 16 mm and 21 mm after the arc opening.
Conclusions
In this study, in order to verify if the preheating influences the end of the transient state of heat flow, the microstructure and microhardness of the welded joint were analyses before and after the theoretical limit for the end of the transient state. According to the results, maybe concluded:
1. The microstructures demonstrate that the preheating affects the end of the transient state. But the microhardness curves are not conclusive about this because of the seam 2 had the same behavior of the seam 3 and seam 4 from the theoretical limit.
2. When the quasi-stationary state is achieved, the curves of microhardness become almost linearly constant, with small variations between the microhardness values of the HAZ in relation to the base metal and the melted zone.
3. The results of microstructure and microhardness show that the equations applied were efficient to determinate the theoretical limit for the end of the transient state near to the surface, for welded joint without preheating.
4. The microhardness curves proved that the thickness influences the heat flow and probably the quasi-stationary state may be achieved first than on the surface.
- OLSON, David Leroy, et al. (1993) ASM Handbook. In Welding, Brazing, and Soldering. Vol. 6. 1ª ed. USA: ASM International, ISBN 978-0-87170-382-8. Cap II. p. 22 – 55.
- KOU, Sindo (2002) Welding metallurgy (2ª edn) United States of America, Wiley-Interscience, ISBN 0-471-43491-4.
- QUITES, Almir M (2008) Metalurgia na Soldagem dos aços. 1ª ed. Florianópolis - Brasil: Soldasoft, ISBN 978-85-8944-505-4.
- MODENESI, Paulo J, MARQUES, Paulo V, SANTOS, Dagoberto B (2012) Introduction to Welding Metallurgy. Belo Horizonte, Brazil: UFMG (Federal University of Minas Gerais), Department of Metallurgical and Materials Engineering.
- WAINER, Emilio, BRANDI, Sergio Duarte, MELLO, et al. (2000) Welding: Processes and Metallurgy. 1st. ed. Sao Paulo, Brazil: Edgard Blücher, ISBN 978-85-2120-238-7.
- MARQUES, Paulo V, MODENESI, Paulo J, BRACARENSE Alexandre Q (2009) Welding fundamentals and technology. 3rd ed. Belo Horizonte, Brazil: UFMG (Federal University Minas Gerais) ISBN 978-85-7041-748-0.
- MODENESI, Paulo José (2011) Weldability of Some Metallic Alloys. Belo Horizonte, Brazil: UFMG (Federal University of Minas Gerais), Department of Metallurgical and Materials Engineering.
- LIPPOLD, John C (2015) Welding Metallurgy and Weldability (1° edn) Ohio, United States of America: John Wiley and Sons ISBN 978-1-118-23070-1.
- CRUZ NETO (2018) Rubelmar Maia de Azevedo - Cooling rate in welding: a new understanding. Polytechnic School of the University of São Paulo. São Paulo Brazil.
- Arruda NF, et al. (2019) Welding and Inspection. São Paulo, Influence of the transient regime on microhardness and microstructure in ASTM-A36 and SAE-1045 steels welded by the MAG process.
- CARVALHO (2014) Robson Lopes de – Influence of quasi-stationary energy on weld bead morphology. Accessible at the Library of the Faculty of Technology of Pindamonhangaba, Pindamonhangaba - SP, Brazil.
- COLPAERT, Humbertus (1974) Metallography of Common Steel Products.4th ed. São Paulo, Brazil: Edgard Blucher, ISBN 978-85-2120-449-7.
- VOORT, George FV (2007) Metallography: Principles and practice (1ª edn) New York, USA: ASM International, ISBN 978- 00-87170-672-0.
- GRONG, Φystein (1997) Metallurgical Modelling of Welding (2ª edn) Trondheim, Norway: Norwegian University of Science and Technology, Department of Metallurgy, ISBN 978-18-6125- 036-0.
- Omer Eyercioglu et al. (2017) – Metals. Switzerland: MDPI, The 2D Finite element microstructure evaluation of V-shaped arcwelding of AISI 1045 steel.
Figures at a glance