Functional Recovery After Bone Marrow Derived Stem Cells in Stroke- A fMRI & Growth Factor Correlation
Received Date:July 23, 2020 Accepted Date: August 17, 2020 Published Date: August 19, 2020
doi: 10.17303/jscr.2020.2.101
Citation: Ashu Bhasin (2020) Functional Recovery After Bone Marrow Derived Stem Cells in Stroke- A fMRI & Growth Factor Correlation. J Stem Cell Rep. 1: 1-10.
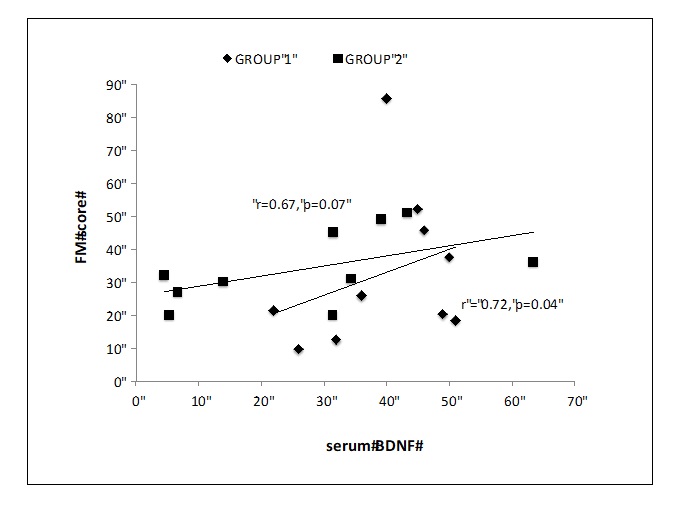
Introduction
Stem Cell research act as a panacea for sequelae after stroke and has been extensively investigated as one of the most promising treatment door [1,2]. Great optimism has resulted from bone marrow-derived stem cells (BMSC) ever since the reestablishment of functionality of injured organs was postulated successfully [3]. Mononuclear stem cells (MNC) have experimented ethically but none of the reports could yield a statistically significant difference in the outcome measures in stroke [4,5].
These cells are capable of producing broad-spectrum cytokines, chemokines, growth factors, and ECM molecules [6,7]. The secreted factors promote cell proliferation and cytoprotection through the production of antioxidants and anti-apoptotic molecules [8]. Two of the most prominent growth factors; brain derived neurotrophic growth factor (BDNF) & vascular endothelial growth factor (VEGF) promote neuronal differentiation, synaptic plasticity, and axonal sprouting enhancing sensorimotor recovery [9].
Imaging technologies are essential for stem cell tracking in-vivo in both experimental and clinical studies. Functional MRI (fMRI) acts as a bio surrogate marker that measures neural activity for studying functional improvement post stroke [10]. The fMRI response can be evoked by direct modulation of the nervous system using physical therapy, pharmacological agents, sensory stimuli like artificial intelligence, cognitive tasks, and the very recent cell transplantation. Stem cells act via two gate theory; direct tissue integration or by paracrine effects [12]. Although the exact mechanisms are not known, some of the explored possibilities are; activation of alternative, already existing or dormant neuronal networks, rewiring of new circuits, and neuroplastic reorganizations [11].
This research is an approach to improve our understanding regarding mechanisms underlying functional recovery of cell therapy and the neurobiological correlates of hand movement coupled with growth factor estimation. The primary objective was to study the efficacy of mononuclear stem cells through functional imaging with growth factor estimation after stroke and safety, feasibility being the secondary objective. We present here functional imaging and growth factor estimation at 8 weeks.
Methods
The inclusion criteria are the same as our last published paper from this RCT [9]. Patients diagnosed with ischemic stroke from 3 months to 2 years of index event with the power of hand muscles at least 2, Brunnstrom stage of recovery: II-IV, NIHSS (National Institute of Health stroke scale) of between 4 and 15, conscious/comprehendible were recruited between Nov 2016-Aug 2018. Twenty (n=20) age-matched individuals acted as healthy controls. We screened 40 patients, 10 refused to participate in the trial and 6 had deranged baseline laboratory values, hence twenty-four patients (n=24) were randomized to two groups. Autoimmune disorders, immune-compromised subjects, chronic liver, and renal failure, progressive neurological worsening, neoplasia contraindication to MRI, and pregnancy formed the exclusion. Patients were randomized (computer-assisted) into two groups; one group receiving intravenous MNC and other placebo/ saline infusion. All patients were administered 8 weeks of neuromotor rehabilitation. The groups were referred to as group 1 & group 2 without treatment allocation being disclosed. Patients were screened, educated about stem cells & bone marrow aspiration technique. Written informed consent, complete medical history, examination, and baseline laboratory tests were performed. The patients were examined by a neurologist and neuro physiotherapist for clinical and functional assessment [9,13,14]. The outcome measures were MRC scale, tone (Ashworth), fuglmeyer score (FM) for upper limb, Brunnstrom stage of stroke recovery, modified Barthel index (mBI) & Edinburgh scale of handedness. The study was approved by ICSCRT (Institute committee for stem cell research and therapy) and is registered with CTRI (CTRI/2014/09/005028). Safety and efficacy endpoints were assessed at 7th day, 8 weeks, 6 months, and one year of stem cell transplantation. Serum growth factors (VEGF and BDNF) & fMRI were measured at baseline, 8 weeks, 6 months, and one year. The clinical outcome measures were blinded to one of the assessors.
BOLD activation pattern
Block design with alternate baseline and activation task with a total of 90 whole-brain EPI measurements (TR =4520 ms, TE=44 ms, slices =31, slice thickness=4 mm) formed the fMRI protocol on 1.5 T MR system (Avanto M/s Siemens, Erlangen, Germany) using a head coil. Ten age-matched healthy controls underwent imaging for comparison with baseline stroke data [15].
Functional MRI Motor task
A self-paced small hand muscle activation motor task was given to patients (minimum 0.5Hz) i.e., fist clenching/extension of the wrist/extension of the affected hand. This was followed by bilateral hand movement. If a mismatch between the target and actual performance or artifact was observed, the measurement was repeated. Adequate rest was provided between the two tasks.
Post-processing
fMRI data were analyzed using SPM8 software (Welcome Department of Cognitive Neurology, London, UK) running under the MATLAB environment (Math works). The functional images were realigned, normalized, and then smoothed by a 6-mm Gaussian filter. The design matrix was created with MNI (Montreal Neurological Institute) coordinates with the number of cluster counts activated. These MNI coordinates were converted to Talairach coordinates and correlated with atlas to get the anatomical location in the brain. The volume of the lesion was analyzed using J-IMAGE software (NIH) [9].
High-resolution T1-weighted structural MR images were spatially co registered into Talairach space and used to generate ROIs for each individual subject. ROIs were created for the primary motor area (M1), premotor cortex (PMC), and supplementary motor area (SMA) based on anatomical definitions of the functional areas. Activation clusters within each ROI were measured in bilateral motor regions using an uncorrected threshold of P < .001. The lateralization of cortical activity during the affected hand pinch was calculated from the number of active voxels in each ROI bilaterally. The LI was calculated as (I−C ) / (I+C), where I is the active voxel count for the specified region in the ipsilesional hemisphere and C is the active voxel count for the region in the contralesional hemisphere while performing the task with the affected hand. LI values range between –1.0 and +1.0, where +1.0 indicates that all the cortical activity occurred within the ipsilesional ROI and –1.0 indicates that all the cortical activity occurred within the contralesional cortical motor area.
Results
Clinical
No serious adverse events were reported in the study until the first follow up. The baseline and clinical characteristics of all patients are presented in table 1. To make results readable, we denoted the groups as 1& 2 without the treatments being disclosed (RCT design). There was no significant difference in clinical scores between group 1 and group 2 at 8 weeks in mBI (65.4 versus 63.2; p=0.72), FM(39.4 vs 41;p=0.53), ashworth (1 vs 2; p=0.29) & MRC (3 vs 2; p=0.17) where as pre and post-treatment scores were statistically significant for both groups 1& 2 respectively (p< 0.05). For VEGF, the baseline level was 336±78.6 pg/ml in group 1 and 370±91.3 pg/ml in group 2 indicating a relatively similar biomarker level at baseline (95% CI~ 5.6 to 3.2; p=0.067). All patients showed improvement pre and post-therapy(p< 0.05), but no statistically significant difference observed between the two groups at 8 weeks (mean 453.5±89.1 vs. 408.4±93.3 pg/ml, 95% CI~13.3–6.7, p=0.67). The median values for VEGF between groups 1 and 2 were also non-significant at 8 weeks (442.1 vs. 400.3 pg/ml, p=0.67).BDNF behaved in a similar path without showing significant changes between groups 1 & 2 (95CI% ~ 5.6 to 7.8; p=0.57) although the mean in group 1 was slightly higher than group 2 (32.8 ng/ml vs 27.3 ng/ml, 95%CI~p=0.57).
Bold
We observed that laterality index of ipsilesional BA 4 & 6 was statistically insignificant between both the groups (95 % CI~ -0.45 to -0.12; p=0.45 and 95% CI~ -0.33 to-0.17; p=0.06 respectively). In group1, LI of BA 4 increased from -0.13 to 0.26 (p=0.003) and LI BA6 increased from -0.06 to 0.43 (p=0.001).In group 2, LI BA4 increased from -0.15 to 0.24 and -0.21 to 0.21 for LI BA6 (table 2). It was observed that there was a 59.2% increase in cluster counts of left BA6 and 56.2% increase in right BA6 in group 2. Similarly, the primary motor cortex (BA4) showed an increase in cluster counts in group1 and 2 with a statistically significant increase in post-treatment (p=0.006). The % signal intensity was nonsignificant between the groups (p=0.43) although it increased from 0.04 to 0.58 in group 1 & 0.04 to 0.36 in group 2. The mean gain in % signal intensity of the motor cortex region was calculated and we observed that group 1 had higher gains than group 2 patients (8.2 vs 5.8, p= 0.67).
Correlation of growth factors with BOLD parameters
Serum BDNF correlated well with FM score at 8 weeks in group 1 (r=0.72, p=0.05) as compared to group 2 (r=0.68,p=0.09) (figure1).VEGF did not show any strong correlation with FM (figure 2) (group 1; r=0.45, p=0.89: group 2; r=0.37, p=0.67); hence we correlated laterality index (LI)of ipsilesional cortex with serum VEGF. Two patients in group 2 had negative LI with a mean VEGF of 430.5 pg/ml, though the correlation line was progressively linear for these patients with positive LI (r=0.53,p=0.12).In group 1, LI showed a positive correlation with serum VEGF in all 10 subjects (r=0.62, p=0.04) with an increasing mean at 8 weeks (figure 3) as compared to group 2 (95% CI~1.4 to 2.3, r=0.58, p=0.56).
Discussion
The novelty of the research is to understand the paracrine mechanisms of stem cells through functional imaging and growth factor up regulation. We reviewed Pubmed, Embase, and Scopus sincerely for stroke recovery and delightfully quote that ours is the first paper to have reported such a phenomenon clinically. The salient conclusion was the safety and feasibility of autologous intravenous MNC in chronic stroke as documented earlier [9,16]. It is an acknowledged fact that the endogenous repair mechanism starts after an acute insult and is approximately completed in the early months. The exogenous recovery continues over a longer period, through cell-based therapies, gene therapy, neuromotor rehabilitation [17].
One of the most fascinating abilities of CNS is to reorganize after any injury. fMRI has provided important insights into the reorganization of the motor cortices after stroke [18,19]. Coupled with cell transplantation, the therapeutics in stroke recovery have been intrigued and well answered by BOLD & DTI. The reported fMRI derived patterns of motor system activation following stroke are variable and their interpretation regarding the recovery process is controversial. We observed an increased number of cluster counts in the motor cortex post-therapy in both groups (group1>group2) which suggests therapy-induced neural plasticity occurring in the brain with cell transplantation and/or exercise regime. Increased BOLD activation was observed in BA6 than BA4 in all patients suggesting the role of BA6 in motor execution, precision, and bimanual hand activation with coordination (figure 4) [20]. To support the hypothesis that chronic stroke patients with less than full recovery are more likely to activate a number of primary, non-primary motor, cingulate and cerebellar regions [21], our results also showed the same trend indicating increased LI of motor cortices (BA 4 & 6) post-treatment in both the groups. The InveST(Intravenous Autologous Bone Marrow Mononuclear Cell Therapy for Ischemic Stroke) trial tested whether an intravenous infusion of autologous bone marrow mononuclear cells between 7 to 30 days (acute to sub-acute) after stroke onset could result in a reduction of the infarct volume and could improve neurological function at day 180 of follow up [22], however, we did not find any change in the lesion volume (data not shown).
The effectiveness of stem cells to enhance functional recovery after stroke has been regularly reported during the last decade in the literature [23]. Another recent phase 2, randomized, placebo-controlled, dose-escalation trial of intravenous multipotent adult progenitor cells showed no difference between the multipotent adult progenitor cell group and placebo groups in global stroke recovery at day 90 (odds ratio 1·08 [95% CI 0·55–2·09], p=0·83) [24]. In a recent report of a regeneration model of spinal cord injury, the C17.2 murine neural progenitor cell line was grafted into the lesion [6,25]. Several growth factors like NGF, BDNF, and GDNF were known to have released as an observation of axonal sprouting of endogenous cells resulting in outcome measures improvement. Another document suggests that functional improvement of animals treated with stem cells after stroke does not take place on the short term, but takes time before the therapeutic effect becomes detectable. This finding is in agreement with previously published works that depict a delayed action of implanted stem cells [26] which justifies our inclusion criteria of patients being selected when the apparent spontaneous recovery after stroke was over and before any scarring occurs in the brain (3mo-2 yrs). Our last paper postulated that the paracrine effects of stem cells levy over cell replacement and this paper is a further exploration of functional recovery post stem cell transplantation after stroke and correlation of growth factors with BOLD imaging [9].
Given the evidence linking BDNF and brain plasticity, research advancements have been made to understand the response of BDNF levels to physical exercise training and its beneficial effects on learning [27]. Thus, using neuro rehabilitative training as an intervention to optimize neuro plasticity and recovery in patients post-stroke has gained considerable interest [28]. Low serum BDNF was found to be associated with poor long-term outcome after stroke [29]. However, the relationship of serum BDNF with the short-term outcome is controversial; one study reported a correlation of low BDNF at 3 months with FIM motor subscore at discharge (r=0.17, p=0.001) and FIM total score at discharge (r=0.15, p=0.004) [30]. However, in our study, we observed that serum BDNF had a positive correlation with fuglmeyer (FM) motor score for upper limb (r=0.72, p=0.05) in group 1as compared to group 2 (figure 1). The task performance was better in both groups at 8 weeks as observed through BOLD imaging with an increase in cluster counts in BA 4 & 6 (p< 0.05). The % signal intensity was non significant between the groups (p=0.43) although it increased from 0.04 to 0.58in group 1 & 0.04 to 0.36 in group 2. Signal intensity is a marker of signal strength of neurons when combined with oxygen to form oxyhemoglobin, an increased signal intensity leads to an enhanced neurovascular coupling consequently neuronal O2 being used for movement [31,32]. Exercise therapy leads to activation of primary motor cortices sensorimotor cortex, premotor cortex, SMA, cingulate, cerebellum, basal ganglia is a unanimous observation, also depicted from our results [33]. Regional blood flow is enhanced by aerobic training exerting its function through neurovascular remodeling mainly through serum VEGF. It did not show a strong correlation with clinical scores in our patients hence we correlated laterality index (LI) of the ipsilesional cortex with serum VEGF in both groups [34]. Also it is known that the compound gets up regulated in the acute phase of stroke [35].
Our a priori intention was to correlate changes in brain activation patterns to changes in performance due to neuro restorative therapies, and not time after stroke and we observed that functional changes in a stroke patient are well correlated with neurotrophic growth hormones and imaging especially BDNF than VEGF [36]. Use of imaging in clinical trials is strongly encouraged to provide as much information as possible to assess vascular/structural lesions, infarct size, cell viability, location, the success, safety of implantation. Imaging should also be used to monitor safety and recovery and, when possible, to investigate mechanisms of action and provide information on surrogate markers of treatment effect. Imaging measures might also be useful to help stratify patients at baseline [37].
To summarize, our interim analysis of a randomized controlled trial suggest that functional recovery post cell transplantation occurs as both the groups performed well on imaging and laboratory parameters. The increase in cluster counts of primary motor cortex along with the upsurge of neurotrophic hormones VEGF & BDNF in both groups indicates therapy-induced reorganization [38,39]. The data is still under evaluation, the study groups will be revealed once the trial is over and a better understanding of the results will be published.
Conclusion
Autologous bone marrow-derived mononuclear stem cells is safe and feasible in chronic stroke. There is a probable positive correlation between the BOLD-signal size, activation of primary &supplementary motor area, and good functional status from sub acute to the chronic period of stroke. Stem cells and neural rehabilitation lead to an increase in neurotrophic hormones and functional reorganization in motor cortices.
Acknowledgment
AB & MVP designed the manuscript. SSK laid the BOLD protocol and fMRI data analysis. SV, SS, NK helped in growth factor correlation, RB & SM helped in revision.
Declaration of conflicting interests
The author(s) declared no potential conflicts of interest with respect to the research, authorship, and/or publication of this article.
Funding
The research was sanctioned by the Department of Science and Technology, DST, India, New Delhi.
- Chang YC, Shyu WC, Lin SZ, Li H (2007) Regenerative therapy for stroke. Cell transplant 16:171-181.
- Koh SH, Park HH (2017) Neurogenesis in stroke recovery. Trans stroke res 8:3-13.
- Wechsler L, Kenmuir CL (2017) Update on cell therapy for stroke. Stroke & Vascular Neurology 2:e: 59-63.
- Prasad K, Sharma A, Garg A, Mohanty S, Bhatnagar S, Johri S et al. (2014) Intravenous autologous bone marrow mononuclear stem cell therapy for ischemic stroke: a multicentric, randomized trial. Stroke 45: 3618-3624.
- Rikhtegar R, Yousefi M, Dolati S, Kasmaei HD, Charsouei S et al. (2019) Stem cell-based cell therapy for neuroprotection in stroke: A review. J Cell Biochem. 120: 8849-8862.
- Lu P, Jones LL, Snyder EY, Tuszynski MH (2003) Neural stem cells constitutively secrete neurotrophic factors and promote extensive host axonal growth after spinal cord injury. Experimental Neurology 181:115-129.
- Baraniak PR, McDevitt TC (2010) Stem cell paracrine actions and tissue regeneration. Reg Med 5: 121-143.
- Burden TJ Paul A, Noisuex N, Prakash H, Sum Tim D (2011) Bone Marrow Stem Cell-Derived Paracrine Factors for Regenerative Medicine: Current Perspectives and Therapeutic Potential. Bone Marrow Res. 207326.
- Bhasin A, Srivastava MVP, Mohanty S, Vivekanandhan S, Sharma S, et al. (2016) Paracrine Mechanisms of Intravenous Bone Marrow-Derived Mononuclear Stem Cells in Chronic Ischemic Stroke. Cerebrovasc Dis Extra 6: 107-119.
- Calautti C, Baron JC (2003) Functional neuroimaging studies of motor recovery after stroke in adults: a review. Stroke 34:1553-1566.
- Stroemer P, Patel S, Hope A, Oliveira C, Pollock K, et al. (2009) The Neural Stem Cell Line CTX0E03 Promotes Behavioral Recovery and Endogenous Neurogenesis After Experimental Stroke in a Dose-Dependent Fashion. Neuro rehabil Neural Repair 23: 895-909.
- Gavins FN, Smith HK (2015) Cell tracking technologies for acute ischemic brain injury. J Cereb Blood Flow Metab. 35: 1090-1099.
- Ismail S, Bilger Y, Evren Y, Rdvani A (2009) Brunnstrom recovery stage and motricity index for the evaluation of upper extremity in stroke: analysis for correlation and responsiveness. Int J Rehabil Res. 32: 228-231.
- Oldfield RC (1972) The assessment and analysis of handedness: the Edinburgh inventory. Neuropsychologia 9: 97-113.
- Bhasin A, Srivastava MVP, Kumaran SS, Bhatia R, Mohanty S (2012) Neural interface of mirror therapy in chronic stroke: a functional imaging study. Neurol India 60: 570-576.
- Bhasin A, Srivastava MVP, Kumaran SS, Mohanty S, et al. (2018) Autologous mesenchymal stem cells in chronic stroke. Cerebrovasc Dis Extra. 2011;1:93-104.
- Cramer SC. Treatments to Promote Neural Repair after Stroke. J Stroke 20: 57-70.
- Rossini PM, Calautti C, Pauri F, Baron JC (2003) Poststroke plastic reorganization in the adult brain. Lancet Neurol 2: 493-502.
- Ward NS, Brown MM, Thompson AJ, Frackowiak RS (2003) Neural correlates of motor recovery after stroke: a longitudinal fMRI study. Brain. 126: 2476-2496.
- Kwakkel G, Kollen B, Lindmann E (2004) Understanding the pattern of functional recovery after stroke: facts and theories. Res Neurol Neuro sci 22: 281-299.
- Fridman EA, Hanakawa T, Chung M, Hummel F, et al. (2004) Reorganization of the human ipsilesional premotor cortex after stroke. Brain. 127: 747-758.
- Prasad K, Sharma A, Mohanty S, Garg A, Bhatnagar S, Johri S, et al. (2014) Intravenous Autologous Bone Marrow Mononuclear Stem Cell Therapy for Ischemic Stroke: A Multi centric, Randomized Trial. Stroke 45: 3618-3624.
- Cabrer PR, Justicia C, Weidermann D, Hoehn N (2010) Stem Cell Mediation of Functional Recovery after Stroke in the Rat. PloS One 5: e12779.
- Hess DC, Wechsler LR, Clarke WM, Savitz SI, Ford GA, Chiu D, et al. (2017) Safety and efficacy of multipotent adult progenitor cells in acute ischaemic stroke (MASTERS): a randomized, double-blind, placebo-controlled, phase 2 trial. The Lancet Neurology 16: 360-368.
- Hiene J, Jason Y, Elisabeth A, Patricia MP, Sarah S, et al. (2003) Transplantation of neurospheres after granule cell lesions in rats: cognitive improvements despite no long-term immunodetection of grafted cells. Behavioral Brain Research 143: 177-191.
- Roitberg B (2004) Transplantation for stroke. Neurological Research 26: 256-264.
- Alcantara CC, Salazar NG, Silva Couto MS, Santos GS, et al. (2018) Post-stroke BDNF Concentration Changes Following Physical Exercise: A Systematic Review. Front Neurol 28: 637-642.
- Cichon N, Bijak M, Czarny P, Miller E, Synowiec E, Slewanki T (2018) Increase in Blood Levels of Growth Factors Involved in the Neuroplasticity Process by Using an Extremely Low Frequency Electromagnetic Field in Post-stroke Patients. Front Aging Neuroscience 10: article 294.
- Stanne TM, Aberg ND, Nilsson S, Jood K, Blomstrand C, Andreasson U et al. (2016) Low circulating acute brain-derived neurotrophic factor levels are associated with poor longterm functional outcome after ischemic stroke. Stroke. 47: 1943- 1945.
- Luo W, Liu T, Li S, Wen H, Zhou F, Zafonte R (2018) The Serum BDNF Level Offers Minimum Predictive Value for Motor Function Recovery After Stroke. Trans stroke Res 3: 10: 342-351.
- Harris JJ, Reynell C, Attwell D (2002) The physiology of developmental changes in BOLD functional imaging signals. Dev Cog Neurosci 1: 199-216.
- Attwell, Iadecola S (2002) The neural basis of functional brain imaging signals. Trends Neurosci. 25: 621-62.
- Ma Y, Qiang L, Man H (2013) Exercise Therapy Augments the Ischemia-Induced Proangiogenic State and Results in Sustained Improvement after Stroke. Int J MolSci 14: 8570–8584.
- Cobbs CS, Chen J, Greenberg DA, Graham SH (1998) Vascular endothelial growth factor expression in transient focal cerebral ischemia in the rat. Neurosci. Lett. 249: 79-82
- Talwar T, Srivastava MVP (2014) Role of vascular endothelial growth factor and other growth factors in post-stroke recovery. Ann indian Acad Neurol 17:1-6.
- Lee HJ, Kim KS, Park IH, Kim SU (2007) Human Neural Stem Cells Over-Expressing VEGF Provide Neuroprotection, Angiogenesis, and Functional Recovery in Mouse Stroke Model. PLoS ONE. 2: 156-162.
- Horei N. Pierreara M, Nizuma K, Sun G, Gill HK, Encarmicion AA (2011) Transplanted stem cell-secreted VEGF effects post-stroke recovery, inflammation, and vascular repair. Stem cells 29.
- M, Zhang Z, Ni A, Dzau VJ (2008) Paracrine mechanisms in adult stem cell signaling and therapy. Circulation Research. 103: 1204-1219.
- Maraei HE, Hassan A, Rizzi R, Althini A, et al. (2018) Potential of Stem Cell-Based Therapy for Ischemic Stroke. Front Neurol 9: 34-39.
Tables at a glance
Figures at a glance